Carbon flows through biological, physical, and geological systems with different processes and at different timescales. The carbon cycle refers to the movement of carbon, in its various forms, from one pool to another (see Figure 1). A carbon pool is any place where carbon can be found, such as plants, the atmosphere, or soil. Carbon pools can also be called stocks or reservoirs. Carbon flux is the process by which carbon moves from one pool to another. For example, carbon moves from the atmosphere to plants through photosynthesis and can move back from plants to the atmosphere through plant or soil respiration during the decomposition process, or during a wildfire when trees and plants are burned.
When a carbon pool absorbs more than it releases, it becomes a carbon sink. For example, the ocean and soil are carbon sinks. Forests can also become carbon sinks. Alternatively, when a carbon pool releases more than it absorbs, it is called a carbon source. Because fossil fuels cannot be created as fast as we are extracting and combusting them, they are considered a carbon source.
The Biological Carbon Cycle
In the biological system, carbon cycles through living organisms, land, water, and the atmosphere by processes including photosynthesis, respiration, and decomposition. Different quantities of carbon reside in each pool, and the carbon moves at different rates between the pools. Some of these processes occur very quickly, while others can take hundreds to thousands of years. For example, some carbon atoms may cycle daily through plant photosynthesis and respiration, while other atoms may be trapped in a tree trunk for hundreds of years. Soil can also trap carbon for long time periods, up to thousands of years.
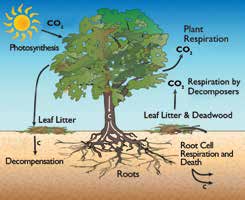
Figure 2. Trees play an important role in the carbon cycle by removing carbon from the atmosphere through photosynthesis. They also release carbon through respiration.
Credit: Valerie Martin, Technical Education Research Centers
Carbon sequestration is the process of transferring atmospheric carbon dioxide into other carbon pools, such as into plant biomass via photosynthesis or into soils from decaying plant and animal matter. Carbon storage in plant biomass is relatively easy to visualize, as plants and composed largely of carbon that they have obtained from atmospheric carbon dioxide through photosynthesis. During photosynthesis, plants convert carbon dioxide and water into sugar in the presence of sunlight. Some of the sugars are used as building blocks to form plant biomass, such as cellulose, lignin, bark, and leaves. Through this process, carbon moves from the atmosphere and is stored in plant cells. Building and maintaining these complex structures requires energy, which the plants create by converting some of the sugars made during photosynthesis into energy through the process of respiration. The process of respiration releases some carbon dioxide back into the atmosphere.
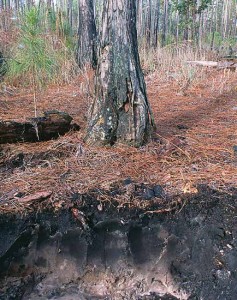
Soils play an important role in the carbon cycle.
Photo credit: Larry Korhnak, University of Florida
Many people do not realize how important soils are in carbon sequestration. Carbon is a major ingredient of soil, and as Figure 1 indicates, soils contain well over twice the amount of carbon found in all terrestrial plants and animals. As plants grow, die, and decay, some of their carbon is stored in soils. This carbon can build continuously over long periods of time and will likely play a significant role in carbon sequestration efforts. The deep ocean and soils represent very large carbon pools, where carbon can stay for hundreds to thousands of years. On the other hand, the exchange of carbon between the atmosphere and plants, along with the exchange between the atmosphere and the surface ocean, occurs continuously. The amount of carbon flowing through the biological system without human impact (through plants and animals to soil and atmosphere) is fairly stable and is considered to be in balance. In Figure 1, the biological fluxes in terrestrial and marine systems account for all their carbon.
For educational materials to support The Carbon Cycle, see the Additional Resources section for this activity.
How Much Carbon Is In a Tree?
The amount of carbon that an individual tree contains depends on tree species and tree size. To calculate carbon storage, foresters and scientists first measure a tree’s height and diameter. Often measurements are taken of trees within a sample plot that is representative of the larger forest, and then the average of these data is used to estimate the biomass in the larger forested area. Tree diameter can be calculated by measuring the tree’s circumference with a measuring tape and dividing this number by pi (π, or 3.14). Because diameter can vary with tree height, measurements are taken at a standard height from the ground—1.5 meters (4.5 feet). This is called diameter at breast height, or DBH. To measure the height of a tree, foresters use instruments called clinometers to calculate tree height. The height of a tree can also be estimated using a ruler. Together, tree height and diameter are used to estimate the tree’s weight. The weight of a living tree is called the green weight and includes both the biomass of the tree and all the water in the tree. The dry weight is calculated by subtracting the weight of the water, which is about 50 percent of a living tree. In general, the amount of carbon stored in an individual tree is about half of the tree’s dry weight.
Carbon Storage vs. Carbon Sequestration
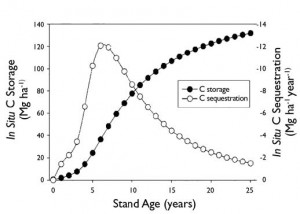
Figure 3. Carbon storage and carbon sequestration for a managed loblolly pine forest in Florida.
Credit: Carlos Gonzalez, University of Florida
Stored carbon is the amount of carbon that exists in a tree’s leaves, wood, stem, roots, and bark at a particular point in time. Because older trees are larger than younger trees, they are able to store more carbon (see “C storage” line in Figure 3). Knowing the amount of carbon stored in a tree and the tree’s age allows us to calculate the rate of carbon sequestration. This represents the net intake of carbon over a period of time (for example, in one year or for the average per year over the tree’s lifespan). Because young, growing trees add biomass at a faster rate than older trees, they are sequestering carbon at a faster rate than older trees (see “C sequestration” line Figure 3). Although the age at which maximum carbon sequestration occurs varies with tree species, the general shape of this relationship is similar for all species.
The Geological Carbon Cycle
The geological carbon cycle occurs over millions of years and includes processes such as rock formation, large-scale land shifts (plate tectonics), weathering, dissolution, volcanic eruptions, and fossilization. Fossil fuels— petroleum, natural gas, and coal—are created over hundreds of millions of years as layers of rock, soil, and sediment covers the remains of dead plants and animals. As this organic matter becomes compacted, pressure and heat turn the carbon into long chains of hydrocarbons. Without human intervention, the carbon would mostly remain trapped far beneath Earth’s surface—making fossil fuels a long-term carbon sink. Limestone is another long-term carbon sink. Limestone forms when ocean sediments, containing the shells of marine animals, are compressed and buried under the ocean floor.
It is important to note that these flows—of carbon—occur at different time scales. While the generation of new fossil fuels is an ongoing process, it is a process that takes place on a time scale of hundreds of years. In contrast, people have only been burning fossil fuels and emitting large quantities of carbon since the 1800s.
(Source: Background adapted from Activity 7: Carbon on the Move, and Activity 8: Measuring Carbon Sequestration in Project Learning Tree’s Southeastern Forests and Climate Change Secondary Education Module.)
Discussion Questions
- What is the biological carbon cycle, and how does it differ from the geological carbon cycle?
- What role do trees and forests play in the carbon cycle?
- What are potential effects of releasing carbon that has been stored in geological carbon pools?